Beneficial Effects of Natural Mineral Waters on Intestinal Inflammation and the Mucosa-Associated Microbiota
Keywords
Article abstract
Natural mineral water (NMWs) intake has been traditionally used in the treatment of various gastrointestinal diseases. We investigated the effect of two French NMWs, one a calcium and magnesium sulphate, sodium chloride, carbonic, and ferruginous water (NMW1), the other a mainly bicarbonate water (NMW2) on the prevention of intestinal inflammation. Intestinal epithelial cells stimulated with heat inactivated Escherichia coli or H2O2 were treated with NMWs to evaluate the anti-inflammatory effects. Moderate colitis was induced by 1% dextran sulfate sodium (DSS) in Balbc/J mice drinking NMW1, NWW2, or control water. General signs and histological features of colitis, fecal lipocalin-2 and pro-inflammatory KC cytokine levels, global mucosa-associated microbiota, were analyzed. We demonstrated that both NMW1 and NMW2 exhibited anti-inflammatory effects using intestinal cells. In induced-colitis mice, NMW1 was effective in dampening intestinal inflammation, with significant reductions in disease activity scores, fecal lipocalin-2 levels, pro-inflammatory KC cytokine release, and intestinal epithelial lesion sizes. Moreover, NMW1 was sufficient to prevent alterations in the mucosa-associated microbiota. These observations, through mechanisms involving modulation of the mucosa-associated microbiota, emphasize the need of investigation of the potential clinical efficiency of such NMWs to contribute, in human beings, to a state of low inflammation in inflammatory bowel disease.
Article content
1. Introduction
2. Results
2.1. Inhibitory Effect of Mineral Waters on Induced Interleukin-8 (IL-8) Secretion by T84 Cells
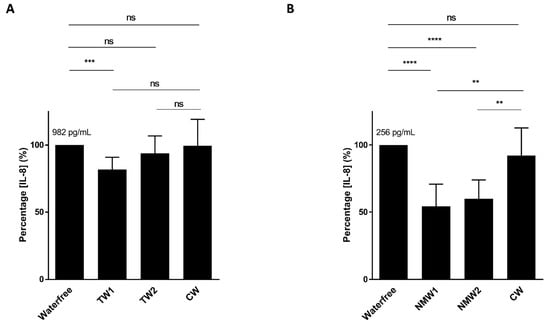
2.2. Mineral Water Decreased the Severity of DSS-Induced Colitis
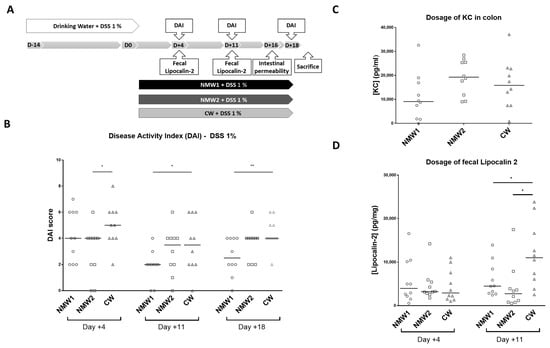
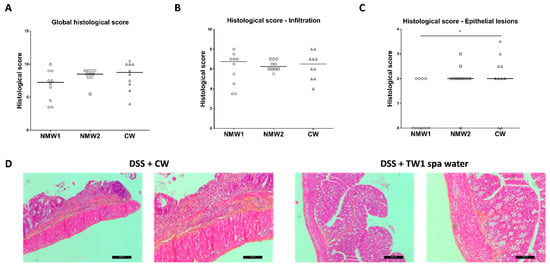
2.3. NMW Treatment Modulates Mucosa-Associated Microbiota in a Mouse Model of Colitis
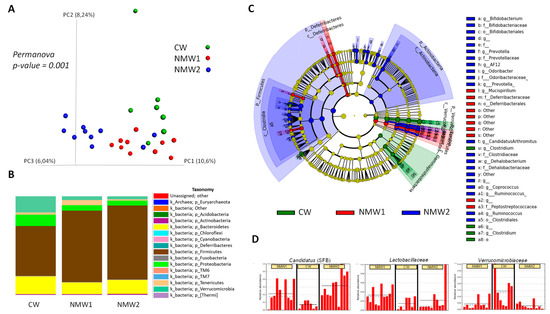
3. Discussion
4. Materials and Methods
4.1. Natural Mineral Waters
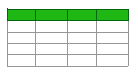
4.2. Intestinal Epithelial Cell Culture and IL-8 Measurement
4.3. Mice and Experimental Protocols
4.4. Clinical Assessment of Colitis
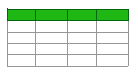
4.5. Histological Evaluation of Colonic Damage
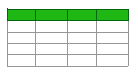
4.6. Quantification of Fecal Lipocalin-2 and Cytokine Release.
4.7. In Vivo Intestinal Permeability Measurement
4.8. Microbiota Analysis by 16S rRNA Gene Sequencing using Illumina Technology
4.9. 16S rRNA Gene Sequencing Analysis
4.10. Statistical Analysis
5. Conclusions
Supplementary Materials
Author Contributions
Funding
Institutional Review Board Statement
Informed Consent Statement
Data Availability Statement
Acknowledgments
Conflicts of Interest
References
- Rocca, G.; Dioni, F.; Rocca, N.; Oliveri, F.; Brunetto, M.R.; Bonino, F. Thermal Care of Functional Dyspepsia Based on Bicarbonate-Sulphate-Calcium Water: A Sequential Clinical Trial. Evid. Based Complement. Altern. Med. 2007, 4, 381–391. [Google Scholar] [CrossRef]
- Gasbarrini, G.; Candelli, M.; Graziosetto, R.-G.; Coccheri, S.; Di Iorio, F.; Nappi, G. Evaluation of Thermal Water in Patients with Functional Dyspepsia and Irritable Bowel Syndrome Accompanying Constipation. World J. Gastroenterol. 2006, 12, 2556–2562. [Google Scholar] [CrossRef] [PubMed]
- Dönmez, A.; Karagülle, M.Z.; Tercan, N.; Dinler, M.; Işsever, H.; Karagülle, M.; Turan, M. SPA Therapy in Fibromyalgia: A Randomised Controlled Clinic Study. Rheumatol. Int. 2005, 26, 168–172. [Google Scholar] [CrossRef]
- Buskila, D.; Abu-Shakra, M.; Neumann, L.; Odes, L.; Shneider, E.; Flusser, D.; Sukenik, S. Balneotherapy for Fibromyalgia at the Dead Sea. Rheumatol. Int. 2001, 20, 105–108. [Google Scholar] [CrossRef] [PubMed]
- Cozzi, F.; Podswiadek, M.; Cardinale, G.; Oliviero, F.; Dani, L.; Sfriso, P.; Punzi, L. Mud-Bath Treatment in Spondylitis Associated with Inflammatory Bowel Disease--a Pilot Randomised Clinical Trial. Jt. Bone Spine 2007, 74, 436–439. [Google Scholar] [CrossRef] [PubMed]
- Ni, J.; Wu, G.D.; Albenberg, L.; Tomov, V.T. Gut Microbiota and IBD: Causation or Correlation? Nat. Rev. Gastroenterol. Hepatol. 2017, 14, 573–584. [Google Scholar] [CrossRef] [PubMed]
- Khan, I.; Ullah, N.; Zha, L.; Bai, Y.; Khan, A.; Zhao, T.; Che, T.; Zhang, C. Alteration of Gut Microbiota in Inflammatory Bowel Disease (IBD): Cause or Consequence? IBD Treatment Targeting the Gut Microbiome. Pathogens 2019, 8, 126. [Google Scholar] [CrossRef] [PubMed]
- Oka, A.; Sartor, R.B. Microbial-Based and Microbial-Targeted Therapies for Inflammatory Bowel Diseases. Dig. Dis. Sci. 2020, 65, 757–788. [Google Scholar] [CrossRef]
- Seksik, P.; Rigottier-Gois, L.; Gramet, G.; Sutren, M.; Pochart, P.; Marteau, P.; Jian, R.; Doré, J. Alterations of the Dominant Faecal Bacterial Groups in Patients with Crohn’s Disease of the Colon. Gut 2003, 52, 237–242. [Google Scholar] [CrossRef]
- Qin, J.; Li, R.; Raes, J.; Arumugam, M.; Burgdorf, K.S.; Manichanh, C.; Nielsen, T.; Pons, N.; Levenez, F.; Yamada, T.; et al. A Human Gut Microbial Gene Catalog Established by Metagenomic Sequencing. Nature 2010, 464, 59–65. [Google Scholar] [CrossRef]
- Darfeuille-Michaud, A.; Boudeau, J.; Bulois, P.; Neut, C.; Glasser, A.L.; Barnich, N.; Bringer, M.A.; Swidsinski, A.; Beaugerie, L.; Colombel, J.F. High Prevalence of Adherent-Invasive Escherichia Coli Associated with Ileal Mucosa in Crohn’s Disease. Gastroenterology 2004, 127, 412–421. [Google Scholar] [CrossRef] [PubMed]
- Sokol, H.; Pigneur, B.; Watterlot, L.; Lakhdari, O.; Bermúdez-Humarán, L.G.; Gratadoux, J.-J.; Blugeon, S.; Bridonneau, C.; Furet, J.-P.; Corthier, G.; et al. Faecalibacterium Prausnitzii Is an Anti-Inflammatory Commensal Bacterium Identified by Gut Microbiota Analysis of Crohn Disease Patients. Proc. Natl. Acad. Sci. USA 2008, 105, 16731–16736. [Google Scholar] [CrossRef] [PubMed]
- Lee, D.; Hong, J.H. The Fundamental Role of Bicarbonate Transporters and Associated Carbonic Anhydrase Enzymes in Maintaining Ion and PH Homeostasis in Non-Secretory Organs. Int. J. Mol. Sci. 2020, 21, 900–908. [Google Scholar] [CrossRef]
- Palileo, C.; Kaunitz, J.D. Gastrointestinal Defense Mechanisms. Curr. Opin. Gastroenterol. 2011, 27, 543–548. [Google Scholar] [CrossRef] [PubMed]
- Seidler, U.E. Gastrointestinal HCO3- Transport and Epithelial Protection in the Gut: New Techniques, Transport Pathways and Regulatory Pathways. Curr. Opin. Pharmacol. 2013, 13, 900–908. [Google Scholar] [CrossRef]
- Tarnawski, A.S.; Ahluwalia, A.; Jones, M.K. Increased Susceptibility of Aging Gastric Mucosa to Injury: The Mechanisms and Clinical Implications. World J. Gastroenterol. 2014, 20, 4467–4482. [Google Scholar] [CrossRef]
- Said, H.; Kaunitz, J.D. Gastrointestinal Defense Mechanisms. Curr. Opin. Gastroenterol. 2016, 32, 461–466. [Google Scholar] [CrossRef] [PubMed]
- Rey, F.E.; Gonzalez, M.D.; Cheng, J.; Wu, M.; Ahern, P.P.; Gordon, J.I. Metabolic Niche of a Prominent Sulfate-Reducing Human Gut Bacterium. Proc. Natl. Acad. Sci. USA 2013, 110, 13582–13587. [Google Scholar] [CrossRef] [PubMed]
- Kushkevych, I.; Cejnar, J.; Treml, J.; Dordević, D.; Kollar, P.; Vítězová, M. Recent Advances in Metabolic Pathways of Sulfate Reduction in Intestinal Bacteria. Cells 2020, 9, 698. [Google Scholar] [CrossRef]
- Levine, J.; Ellis, C.J.; Furne, J.K.; Springfield, J.; Levitt, M.D. Fecal Hydrogen Sulfide Production in Ulcerative Colitis. Am. J. Gastroenterol. 1998, 93, 83–87. [Google Scholar] [CrossRef]
- Pitcher, M.C.; Beatty, E.R.; Cummings, J.H. The Contribution of Sulphate Reducing Bacteria and 5-Aminosalicylic Acid to Faecal Sulphide in Patients with Ulcerative Colitis. Gut 2000, 46, 64–72. [Google Scholar] [CrossRef]
- Wallace, J.L.; Vong, L.; McKnight, W.; Dicay, M.; Martin, G.R. Endogenous and Exogenous Hydrogen Sulfide Promotes Resolution of Colitis in Rats. Gastroenterology 2009, 137, 569–578. [Google Scholar] [CrossRef]
- Dostal, A.; Baumgartner, J.; Riesen, N.; Chassard, C.; Smuts, C.M.; Zimmermann, M.B.; Lacroix, C. Effects of Iron Supplementation on Dominant Bacterial Groups in the Gut, Faecal SCFA and Gut Inflammation: A Randomised, Placebo-Controlled Intervention Trial in South African Children. Br. J. Nutr. 2014, 112, 547–556. [Google Scholar] [CrossRef]
- Kortman, G.A.M.; Mulder, M.L.M.; Richters, T.J.W.; Shanmugam, N.K.N.; Trebicka, E.; Boekhorst, J.; Timmerman, H.M.; Roelofs, R.; Wiegerinck, E.T.; Laarakkers, C.M.; et al. Low Dietary Iron Intake Restrains the Intestinal Inflammatory Response and Pathology of Enteric Infection by Food-Borne Bacterial Pathogens. Eur. J. Immunol. 2015, 45, 2553–2567. [Google Scholar] [CrossRef]
- Kayama, H.; Kohyama, M.; Okuzaki, D.; Motooka, D.; Barman, S.; Okumura, R.; Muneta, M.; Hoshino, K.; Sasaki, I.; Ise, W.; et al. Heme Ameliorates Dextran Sodium Sulfate-Induced Colitis through Providing Intestinal Macrophages with Noninflammatory Profiles. Proc. Natl. Acad. Sci. USA 2018, 115, 8418–8423. [Google Scholar] [CrossRef]
- Vargas-Robles, H.; Castro-Ochoa, K.F.; Citalán-Madrid, A.F.; Schnoor, M. Beneficial Effects of Nutritional Supplements on Intestinal Epithelial Barrier Functions in Experimental Colitis Models in Vivo. World J. Gastroenterol. 2019, 25, 4181–4198. [Google Scholar] [CrossRef]
- Ivanov, I.I.; Atarashi, K.; Manel, N.; Brodie, E.L.; Shima, T.; Karaoz, U.; Wei, D.; Goldfarb, K.C.; Santee, C.A.; Lynch, S.V.; et al. Induction of Intestinal Th17 Cells by Segmented Filamentous Bacteria. Cell 2009, 139, 485–498. [Google Scholar] [CrossRef]
- Ganesh, B.P.; Klopfleisch, R.; Loh, G.; Blaut, M. Commensal Akkermansia Muciniphila Exacerbates Gut Inflammation in Salmonella Typhimurium-Infected Gnotobiotic Mice. PLoS ONE 2013, 8, e74963. [Google Scholar] [CrossRef]
- Mitchell, J.; Kim, S.J.; Koukos, G.; Seelmann, A.; Veit, B.; Shepard, B.; Blumer-Schuette, S.; Winter, H.S.; Iliopoulos, D.; Pothoulakis, C.; et al. Colonic Inhibition of Phosphatase and Tensin Homolog Increases Colitogenic Bacteria, Causing Development of Colitis in Il10-/- Mice. Inflamm. Bowel Dis. 2018, 24, 1718–1732. [Google Scholar] [CrossRef]
- Seregin, S.S.; Golovchenko, N.; Schaf, B.; Chen, J.; Pudlo, N.A.; Mitchell, J.; Baxter, N.T.; Zhao, L.; Schloss, P.D.; Martens, E.C.; et al. NLRP6 Protects Il10-/- Mice from Colitis by Limiting Colonization of Akkermansia Muciniphila. Cell Rep. 2017, 19, 2174. [Google Scholar] [CrossRef]
- Pozsgai, G.; Benkó, R.; Barthó, L.; Horváth, K.; Pintér, E. Thermal Spring Water Drinking Attenuates Dextran-Sulfate-Sodium-Induced Colitis in Mice. Inflammopharmacology 2015, 23, 57–64. [Google Scholar] [CrossRef] [PubMed]
- Eliasse, Y.; Galliano, M.-F.; Redoules, D.; Espinosa, E. Effect of Thermal Spring Water on Human Dendritic Cell Inflammatory Response. J. Inflamm. Res. 2019, 12, 181–194. [Google Scholar] [CrossRef]
- Shiraishi, H.; Fujino, M.; Shirakawa, N.; Ishida, N.; Funato, H.; Hirata, A.; Abe, N.; Iizuka, M.; Jobu, K.; Yokota, J.; et al. Effect of Minerals on Intestinal IgA Production Using Deep Sea Water Drinks. Biol. Pharm. Bull. 2017, 40, 1700–1705. [Google Scholar] [CrossRef]
- Takeuchi, H.; Higuchi, K.; Yoshikane, Y.; Takagi, R.; Tokuhiro, S.; Takenaka, K.; Oboshi, W.; Kimura, A.; Islam, J.M.; Kaneko, A.; et al. Drinking Refined Deep-Sea Water Improves the Gut Ecosystem with Beneficial Effects on Intestinal Health in Humans: A Randomized Double-Blind Controlled Trial. Nutrients 2020, 12, 2646. [Google Scholar] [CrossRef]
- Gaboriau-Routhiau, V.; Rakotobe, S.; Lécuyer, E.; Mulder, I.; Lan, A.; Bridonneau, C.; Rochet, V.; Pisi, A.; De Paepe, M.; Brandi, G.; et al. The Key Role of Segmented Filamentous Bacteria in the Coordinated Maturation of Gut Helper T Cell Responses. Immunity 2009, 31, 677–689. [Google Scholar] [CrossRef]
- Schnupf, P.; Gaboriau-Routhiau, V.; Cerf-Bensussan, N. Modulation of the Gut Microbiota to Improve Innate Resistance. Curr. Opin. Immunol. 2018, 54, 137–144. [Google Scholar] [CrossRef]
- Al Nabhani, Z.; Dulauroy, S.; Marques, R.; Cousu, C.; Al Bounny, S.; Déjardin, F.; Sparwasser, T.; Bérard, M.; Cerf-Bensussan, N.; Eberl, G. A Weaning Reaction to Microbiota Is Required for Resistance to Immunopathologies in the Adult. Immunity 2019, 50, 1276–1288. [Google Scholar] [CrossRef] [PubMed]
- Chen, B.; Chen, H.; Shu, X.; Yin, Y.; Li, J.; Qin, J.; Chen, L.; Peng, K.; Xu, F.; Gu, W.; et al. Presence of Segmented Filamentous Bacteria in Human Children and Its Potential Role in the Modulation of Human Gut Immunity. Front. Microbiol. 2018, 9, 1403. [Google Scholar] [CrossRef]
- Murakami, S.; Goto, Y.; Ito, K.; Hayasaka, S.; Kurihara, S.; Soga, T.; Tomita, M.; Fukuda, S. The Consumption of Bicarbonate-Rich Mineral Water Improves Glycemic Control. Evid. Based Complement. Altern. Med. 2015, 2015, 824395. [Google Scholar] [CrossRef]
- Pedron, R.; Esposito, A.; Bianconi, I.; Pasolli, E.; Tett, A.; Asnicar, F.; Cristofolini, M.; Segata, N.; Jousson, O. Genomic and Metagenomic Insights into the Microbial Community of a Thermal Spring. Microbiome 2019, 7, 8. [Google Scholar] [CrossRef]
- Martin, R.; Henley, J.B.; Sarrazin, P.; Seité, S. Skin Microbiome in Patients With Psoriasis Before and After Balneotherapy at the Thermal Care Center of La Roche-Posay. J. Drugs Dermatol. 2015, 14, 1400–1405. [Google Scholar]
- Casas, C.; Ribet, V.; Alvarez-Georges, S.; Sibaud, V.; Guerrero, D.; Schmitt, A.-M.; Redoulès, D. Modulation of Interleukin-8 and Staphylococcal Flora by Avène Hydrotherapy in Patients Suffering from Chronic Inflammatory Dermatoses. J. Eur. Acad. Dermatol. Venereol. 2011, 25, 19–23. [Google Scholar] [CrossRef]
- Akiyama, H.; Yamasaki, O.; Tada, J.; Kubota, K.; Arata, J. Antimicrobial Effects of Acidic Hot-Spring Water on Staphylococcus Aureus Strains Isolated from Atopic Dermatitis Patients. J. Dermatol. Sci. 2000, 24, 112–118. [Google Scholar] [CrossRef]
- Zeichner, J.; Seite, S. From Probiotic to Prebiotic Using Thermal Spring Water. J. Drugs Dermatol. 2018, 17, 657–662. [Google Scholar] [PubMed]
- Caporaso, J.G.; Lauber, C.L.; Walters, W.A.; Berg-Lyons, D.; Huntley, J.; Fierer, N.; Owens, S.M.; Betley, J.; Fraser, L.; Bauer, M.; et al. Ultra-High-Throughput Microbial Community Analysis on the Illumina HiSeq and MiSeq Platforms. ISME J. 2012, 6, 1621–1624. [Google Scholar] [CrossRef]
- Caporaso, J.G.; Kuczynski, J.; Stombaugh, J.; Bittinger, K.; Bushman, F.D.; Costello, E.K.; Fierer, N.; Peña, A.G.; Goodrich, J.K.; Gordon, J.I.; et al. QIIME Allows Analysis of High-Throughput Community Sequencing Data. Nat. Methods 2010, 7, 335–336. [Google Scholar] [CrossRef] [PubMed]
- Edgar, R.C. Search and Clustering Orders of Magnitude Faster than BLAST. Bioinforma. Oxf. Engl. 2010, 26, 2460–2461. [Google Scholar] [CrossRef]
- McDonald, D.; Price, M.N.; Goodrich, J.; Nawrocki, E.P.; DeSantis, T.Z.; Probst, A.; Andersen, G.L.; Knight, R.; Hugenholtz, P. An Improved Greengenes Taxonomy with Explicit Ranks for Ecological and Evolutionary Analyses of Bacteria and Archaea. ISME J. 2012, 6, 610–618. [Google Scholar] [CrossRef]
- Price, M.N.; Dehal, P.S.; Arkin, A.P. FastTree: Computing Large Minimum Evolution Trees with Profiles Instead of a Distance Matrix. Mol. Biol. Evol. 2009, 26, 1641–1650. [Google Scholar] [CrossRef]
- Lozupone, C.; Hamady, M.; Knight, R. UniFrac—An Online Tool for Comparing Microbial Community Diversity in a Phylogenetic Context. BMC Bioinform. 2006, 7, 371. [Google Scholar] [CrossRef] [PubMed]
- Lozupone, C.; Knight, R. UniFrac: A New Phylogenetic Method for Comparing Microbial Communities. Appl. Environ. Microbiol. 2005, 71, 8228–8235. [Google Scholar] [CrossRef] [PubMed]